Groundwork for spin transistor
We’re used to computer chips increasing in speed and capacity. This is achieved by making transistors smaller, so that more fit on the computer processor. However, miniaturization is nearing its limit. One solution may be transistors that operate on electron spin rather than a charge. Sander Kamerbeek defended his PhD thesis on this topic on 9 September.
Three components determine the speed of a computer: the processor (CPU), the volatile RAM memory and the non-volatile hard-disk storage. ‘And of course the connection between these’, says physicist Sander Kamerbeek. He explains the workings of transistors, which do all the hard work on the CPU. ‘Basically, a current flows through a channel, and a switch known as the gate can open or close that channel.’ If voltage is applied to the gate, the transistor switches between low and high resistance, or in computer terms 1 or 0.
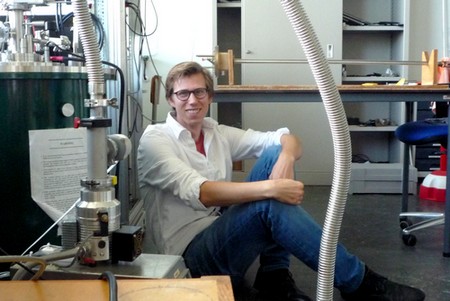
In 1965, Gordon Moore made an observation, known as ‘Moore’s Law’, that the number of transistors per surface unit on integrated circuits doubles every two years. But Moore’s Law failed around 2011. Transistors had become so small that physical constraints prevented further miniaturization. So physicists began looking for new ways to increase the capacity of CPUs.
One way to improve transistors is to change how they work. ‘Right now, they’re based on an electron current’, says Kamerbeek. A current means heat, and the smaller the transistor, the more problematic the heat production.
Stability
An alternative is to design transistors that operate on electron spin, which switch faster, use less energy and produce less heat. Spin is a quantum mechanical characteristic of electrons. ‘To put it simply, spin has two settings: up and down. A few ingredients are needed to build a spin transistor. First, ferromagnetic materials that have a higher resistance for one spin – say down – than the other. Second, a channel to transport the spin. Third, the ability to switch the spin from – say – down to up inside the channel.’
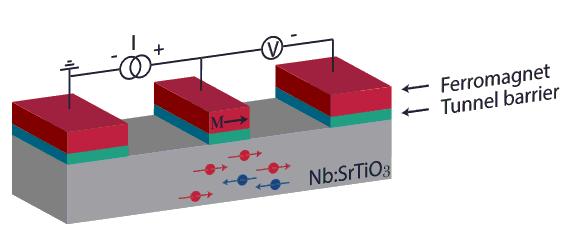
Once you have these components, you can create a flow of up spins in the channel by injecting them in the channel with a ferromagnet. The spins flow through the channel and can be extracted at the other end by another ferromagnet. The ferromagnet senses the spin resistance in the channel: this depends on the spin direction. So if you now use the gate to flip the spin from up to down, or vice versa, the ferromagnet senses a high or low resistance.
Kamerbeek: ‘The problem is to find a material with a stable spin that can also be switched with a voltage by using a gate.’ In contrast to the charge, which is always the same in an electron, the spin can change spontaneously from up to down, or vice versa. ‘For instance, if the electron bumps into an impurity in the material, its spin can unintentionally flip’, says Kamerbeek. In graphene, the two-dimensional form of carbon, the spins are very stable but difficult to switch with a gate. ‘So we looked for a semiconductor with the right combination of stability and switchability.’
Impurities
Strontium titanium oxide was an interesting candidate. Kamerbeek studied this material by making different devices out of it. Theoretical studies have shown that spins could be stable in it and that switching was possible. He observed that voltage could strongly affect the electron spin, which made it a promising contender for a gate to flip the spin direction.
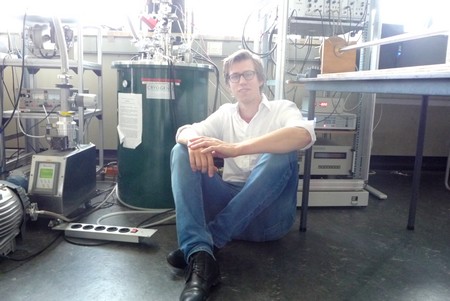
However, the spins in the channel are short-lived. The spin current was transported for only 10 nanometres before it dissipated. ‘This is still a good result, especially since we could influence the spins at room temperature. Others have been able to switch the spin direction at very low temperatures, but you can’t use those in a real world application. But in order to use our material, the spin transport should be around at least a couple of hundred nanometres, so two orders of magnitude more than what we accomplished.’
Has his research brought the spin-driven transistor any closer? That is a difficult question, says Kamerbeek. ‘The spin transistor could very well realize a technological and digital revolution. However, we don’t know if and when such transistors will become a reality. But my work was aimed at understanding if this particular material had interesting properties for creating electronics using the electron spin and what extra functionalities it could offer over conventional semiconductors. From our findings, this material does indeed look promising.
Kamerbeek is continuing his work as a postdoc in the group of his thesis supervisor Tamalika Banerjee.
The supervisor
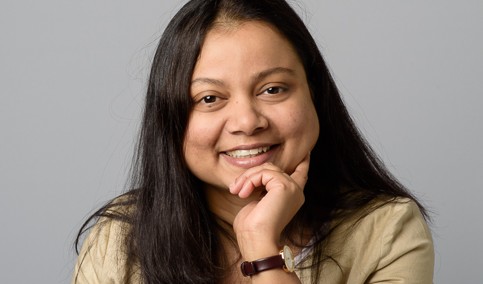
So why is this PhD thesis interesting? Tamalika Banerjee explains. ‘Small and cool, fast and cheap, are oxymorons in today’s trillion-dollar microelectronics industry.’ But this has spurred the growth of alternative technologies such as spintronics that aim to unite these oxymorons with an ambitious integration of new materials, devices and operating principles.
Banerjee has written several research proposals on new materials and phenomena that could be used to build an all-oxide spintronics memory. Alexander Kamerbeek addressed this topic in his thesis. ‘Alexander successfully demonstrated results at room temperature using a simple device geometry and further showed that the manipulation of injected spins could be realized by electric fields – a new approach in spintronics and a vital ingredient for spin logic. In another clever set of experiments, he demonstrated that the well-known electroresistance effects present in such materials can be coupled to spin memory.’ This provides unique prospects for reconfigurable spintronics architecture with this new class of materials. ‘Indeed’, says Banerjee, ‘Promising times lie ahead for electronics beyond Moore, and it may not be long before those four oxymora can be happily united.’
Last modified: | 17 March 2020 2.49 p.m. |
More news
-
10 June 2024
Swarming around a skyscraper
Every two weeks, UG Makers puts the spotlight on a researcher who has created something tangible, ranging from homemade measuring equipment for academic research to small or larger products that can change our daily lives. That is how UG...
-
21 May 2024
Results of 2024 University elections
The votes have been counted and the results of the University elections are in!