Fundamental research with life-size effects
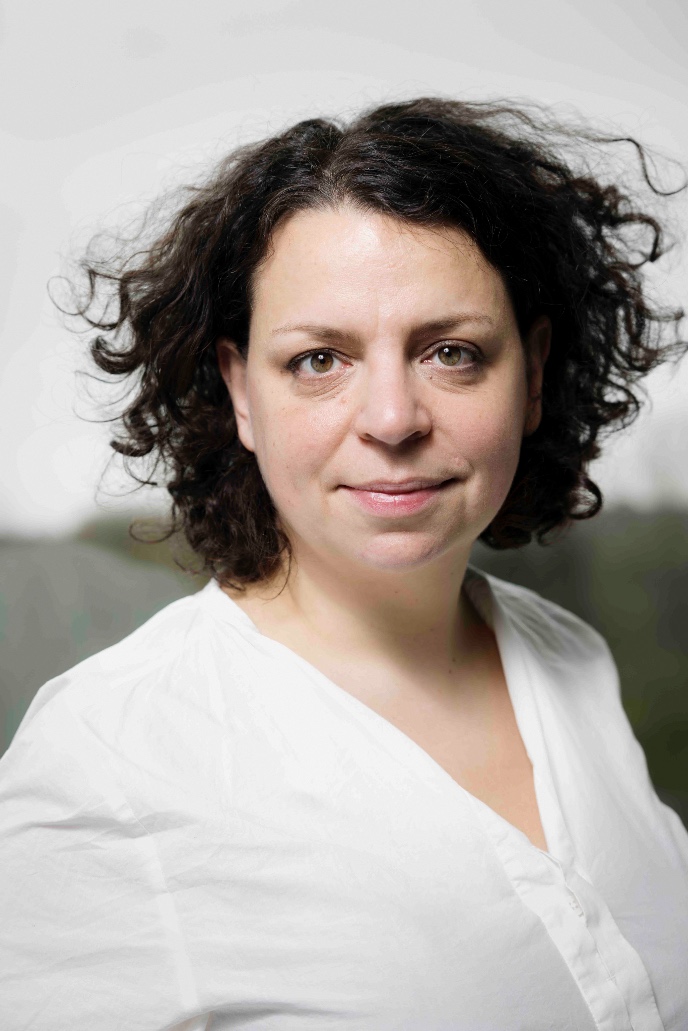
‘I love that I can do truly fundamental research while still contributing to solving major societal problems,’ says Nathalie Katsonis, Professor of Chemistry. She develops adaptive molecular materials and studies the chemical origins of life, which in turn yield insights for vaccines and clearing up oil spills at sea. Today, it was announced that Katsonis will receive the Ammodo Science Award for Fundamental Research.
FSE Science Newsroom | Text Charlotte Vlek | Images Leoni von Ristok
Imagine a man-made material that pops open when light shines on it, like the seedpod of an orchid. Or a material that winds or unwinds like the tendrils of a climbing cucumber plant, or one that follows the sun, just like a sunflower. These kinds of active materials are what Katsonis is developing in her lab. ‘We need to radically change how we think about materials,’ she states. ‘The green materials of the future will have to be able to change and adapt to their environment, instead of fighting against it. These materials will also be recyclable, so we stop throwing away old materials as soon as we have invented something new.’
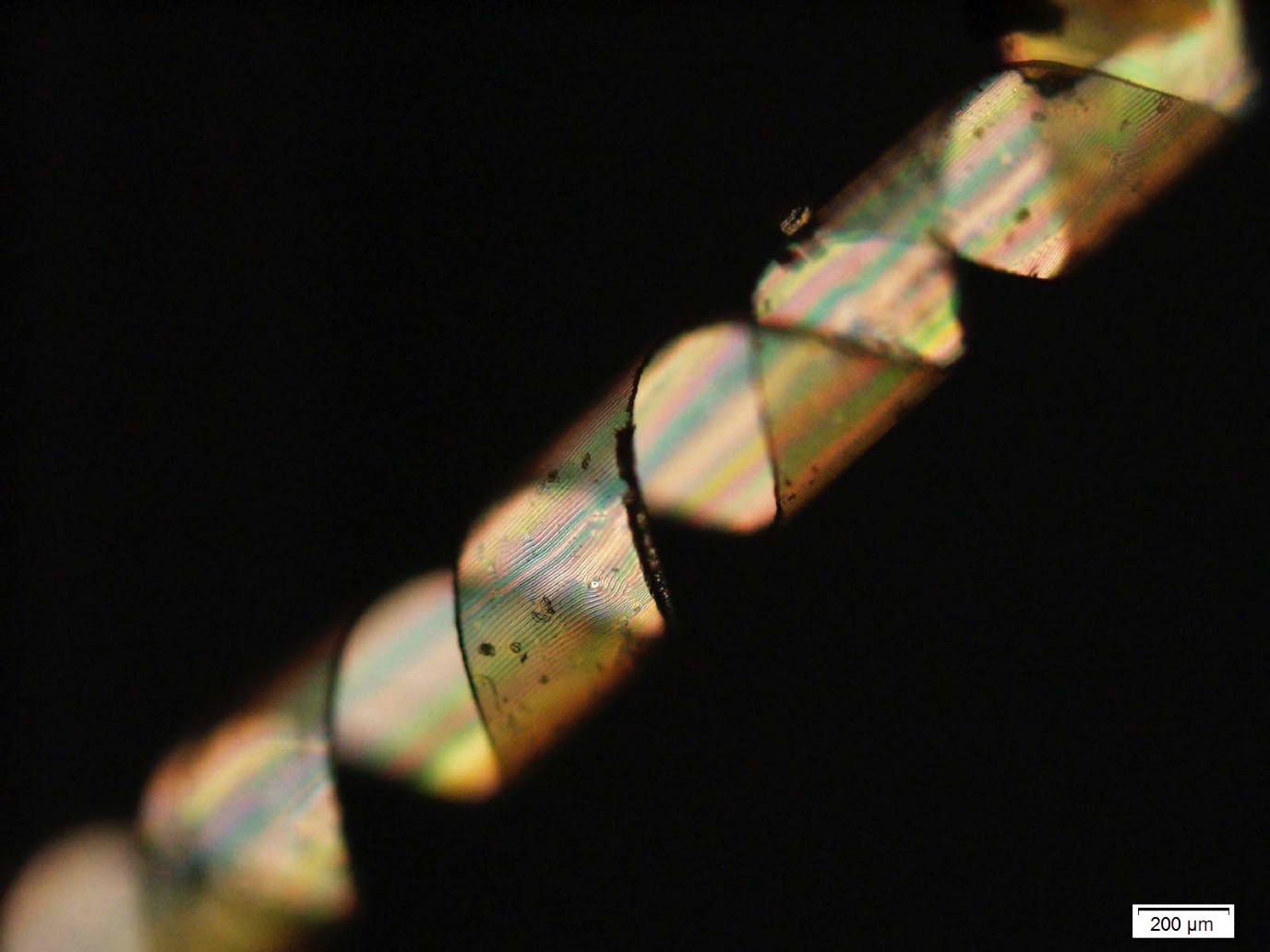
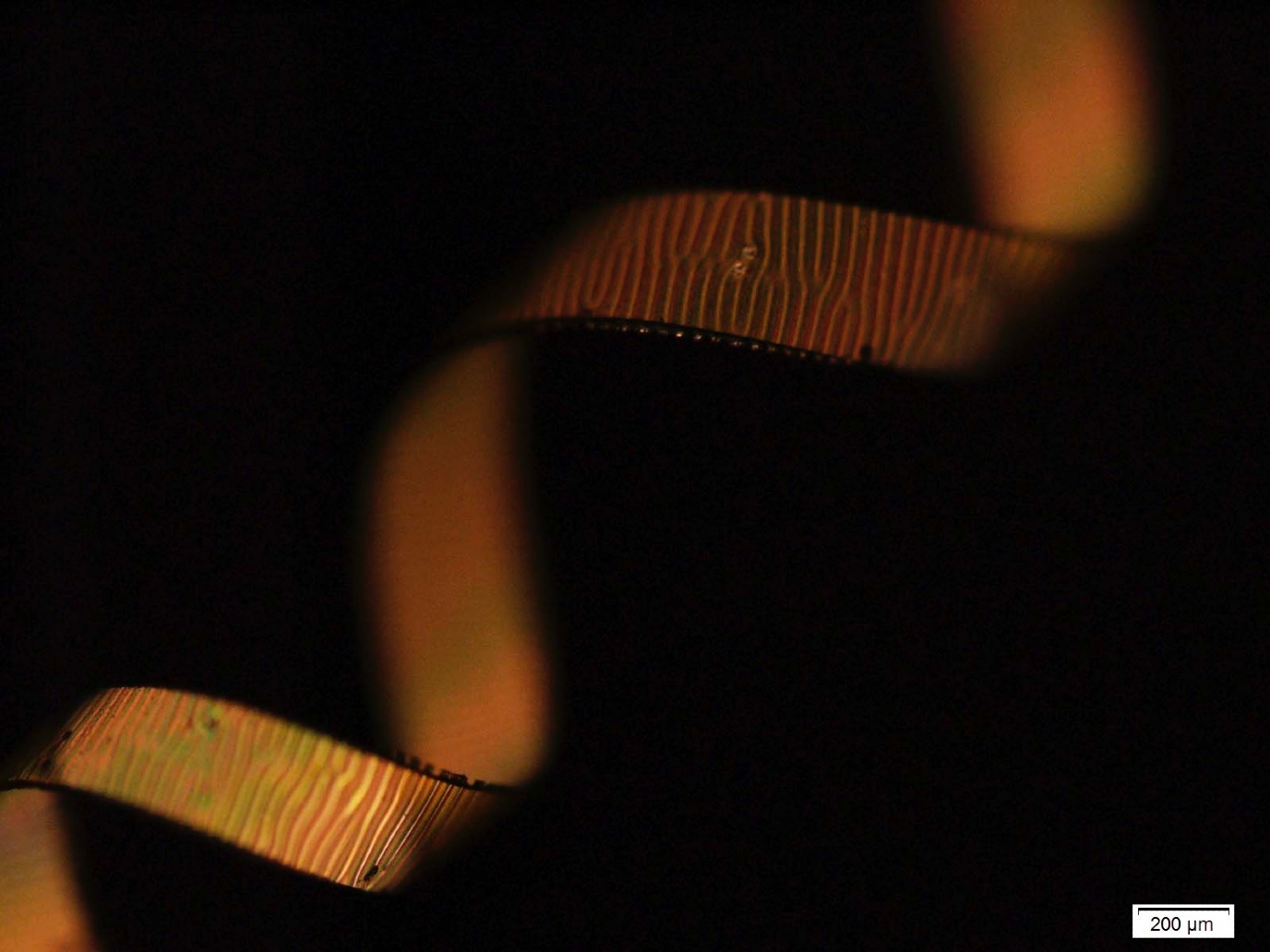
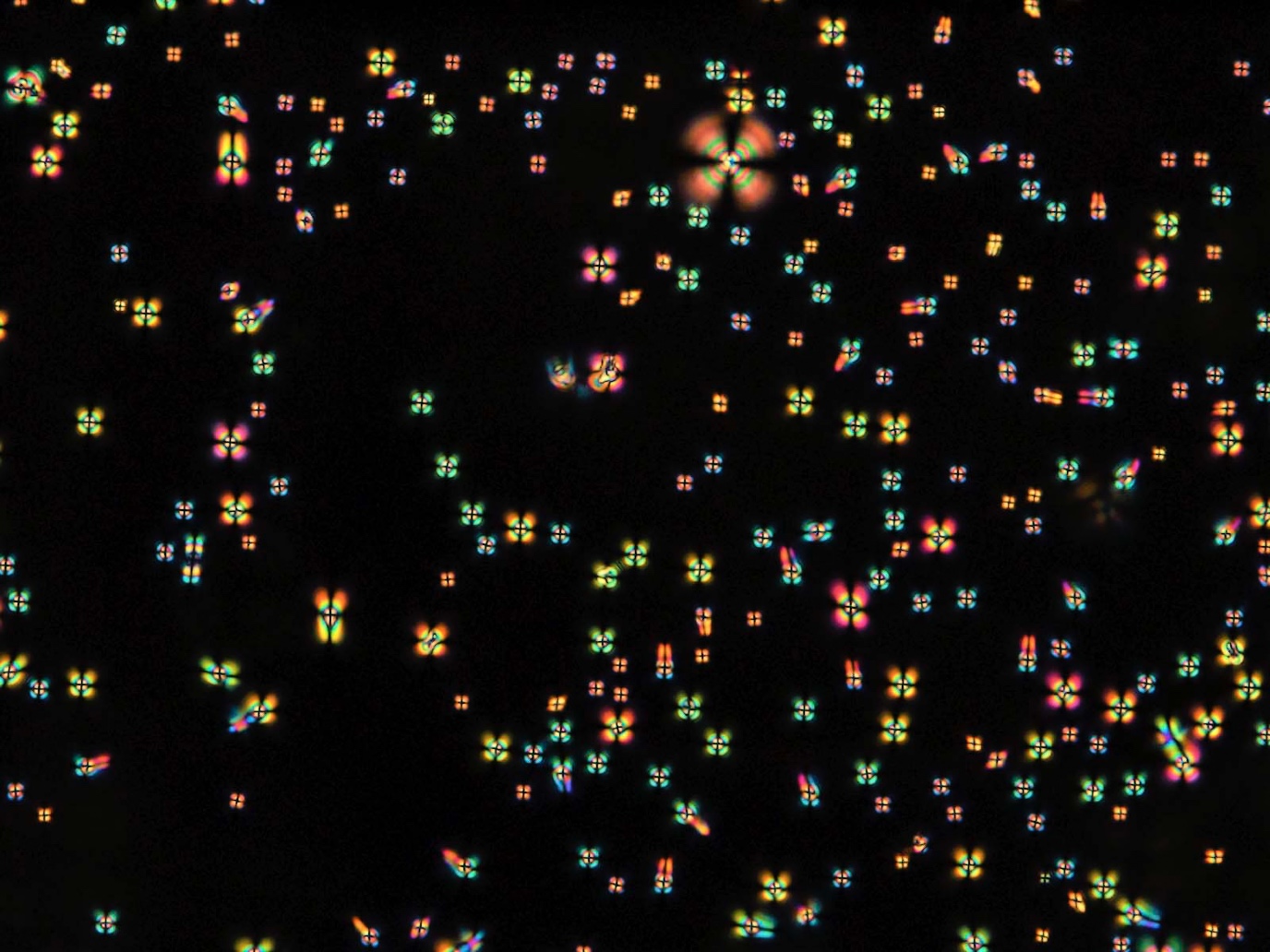
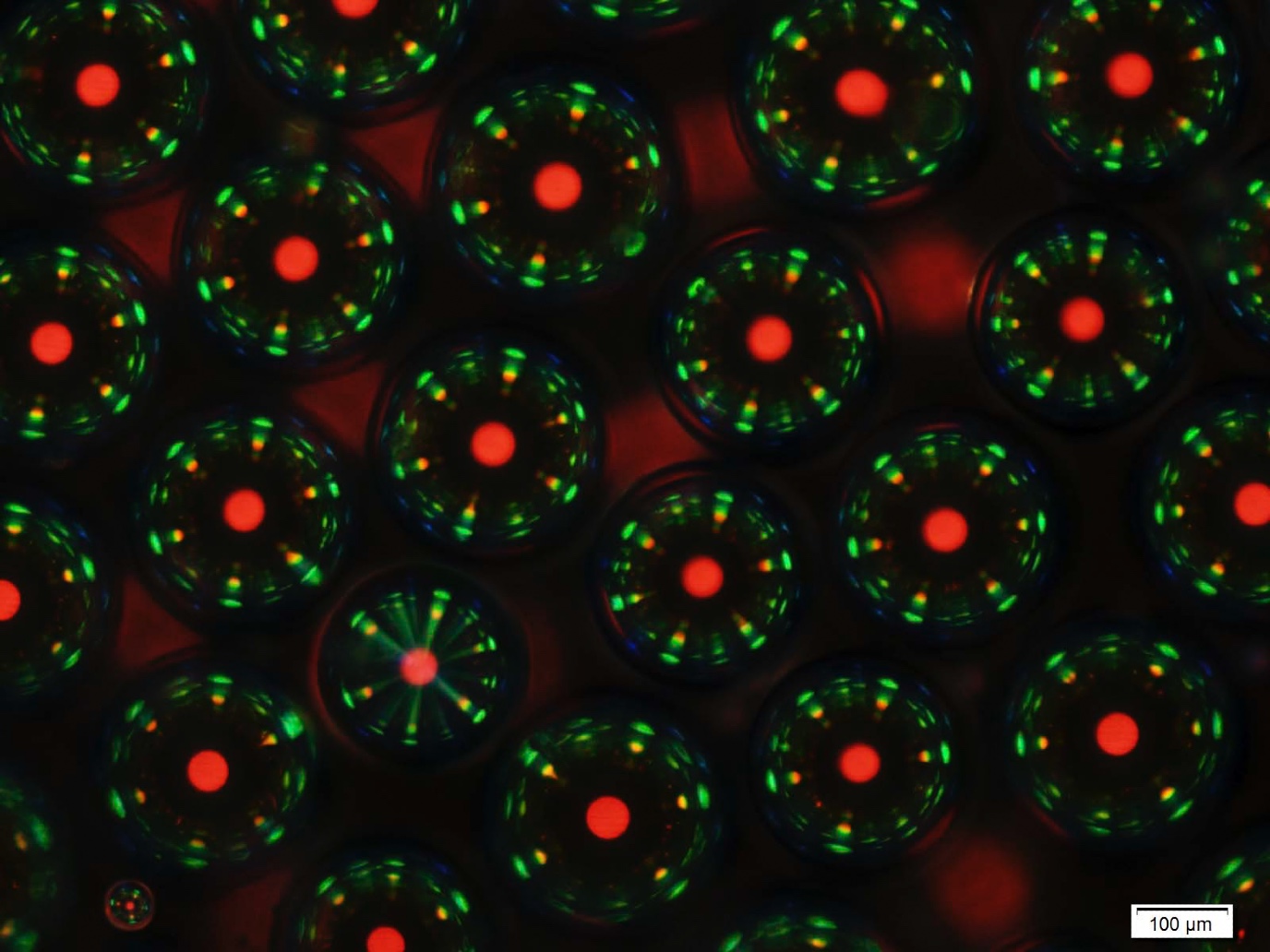
Katsonis develops adaptive materials by incorporating artificial molecular machines into larger molecular ensembles. Molecular machines are man-made molecules that display sophisticated movement or exert a force in response to external stimuli, such as light. Building on her extensive expertise in the field, she previously worked as a postdoctoral fellow with Ben Feringa, who was awarded the 2016 Nobel Prize for his pioneering work on artificial molecular machines, including the nanocar: a molecular machine powered by four molecular motors as wheels.
From nano to macro
When a molecular machine operates and moves, the movement occurs at the nanoscale. I integrate molecular machines into larger ensembles that can move and change their shape at larger scales
‘When a molecular machine operates and moves, the movement occurs at the nanoscale,’ Katsonis explains. ‘I integrate molecular machines into larger ensembles, and eventually into materials, that can move and change their shape at larger scales. The challenge is to have all these machines move in sync: at the same moment and all in the same direction.’ To make this happen, Katsonis designs the material to have an inherent ‘twist’ in it. She uses a property of molecules called chirality—where a molecule and its mirror image, like left and right hands, cannot be superimposed. In nature, living systems often prefer one mirror image over the other, and that tiny choice can scale up, creating preferred directions in the way materials are organized.
As in nature, by using only one ‘handedness’, Katsonis was able to create a material that tends to twist in a certain direction once the molecular motors come into action, instead of dispersing their actions chaotically and in all directions. By combining engineering principles from plants—such as molecular organization—with those from animals, like the bio-molecular machines found in muscles, Katsonis has developed unprecedented active materials.
Chirality in nature
Chirality, the possibility of left- and right-handedness in molecules, appears to be quite important in nature. It has to do with the specific organization of atoms in 3D that can result in two identical molecules that are mirror images of each other. And yet, just like with an unsuccessful handshake with a right hand and a left hand between two persons, these mirrored molecules will not behave the same.
The human body is also extremely sensitive to chirality. A left-handed molecule can smell completely different from its right-handed counterpart. An example of this is S-carvone: an oil that can be found in caraway and dill, which smells like caraway seeds, while the mirror image of the same molecule smells like spearmint. ‘There are a lot of curious things related to chirality,’ Katsonis reports. ‘For instance, a DNA helix always twists in the same direction, as do galaxies.’
Inspiration from nature
Katsonis often draws inspiration from nature, because biological materials are simultaneously functional, adaptive, and renewable. Just look at the leaves of a tree, which can convert sunlight into nutrients, but can also turn into compost. ‘All that lives, moves,’ Katsonis states, and she does not mean that trees are secretly able to move to a different spot in the forest overnight. Rather, all of life continuously grows or changes shape. And with it comes an inherent ability to adapt. As Leonardo da Vinci once wrote: ’Motion is the cause of all life’.
The practical applications of the origins of life
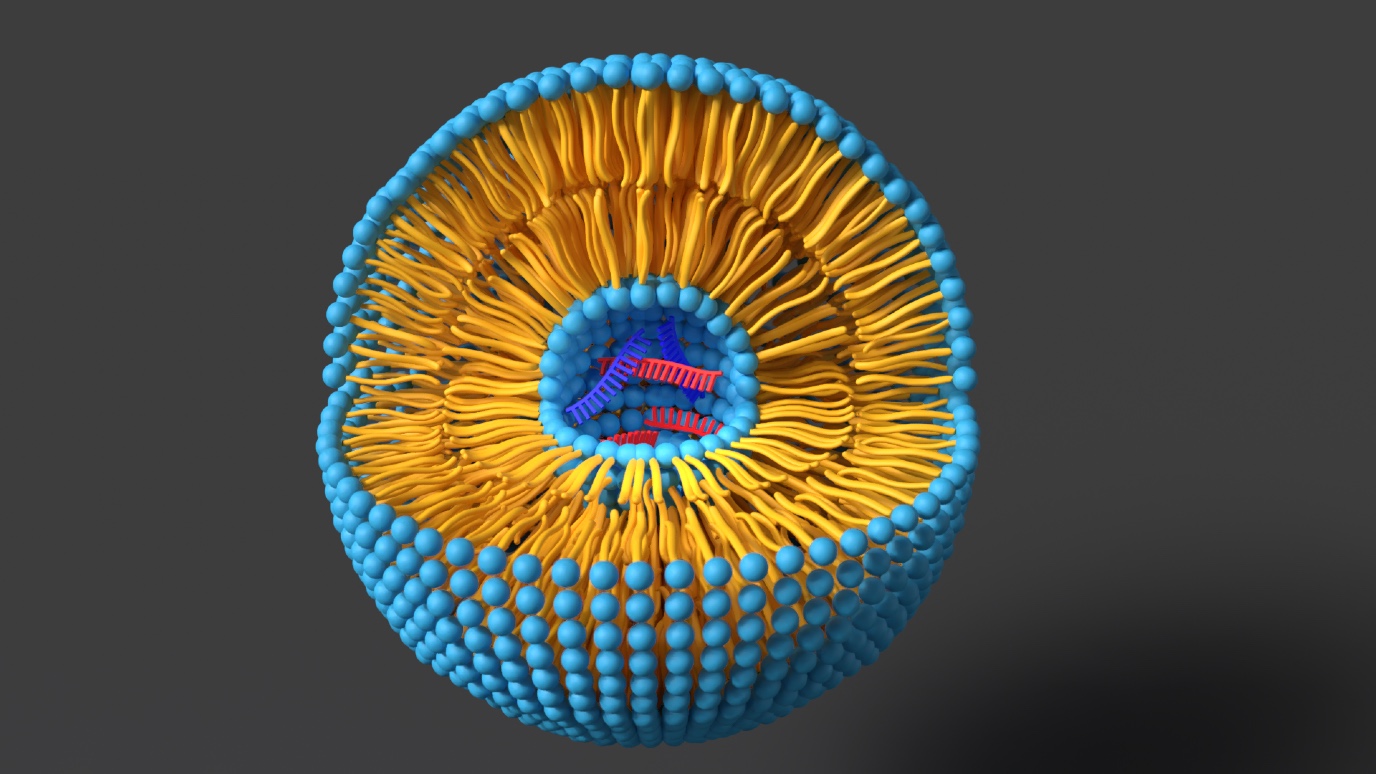
Katsonis’ most recent research dives into the molecular origins of life. Researchers across the world agree that life must have started with a string of genetic information (short strands of RNA) encapsulated in a little compartment to form a primitive cell. ‘RNA is the ultimate molecular machine,’ says Katsonis: ‘A molecule that codes information, that can copy itself, and that can make other reactions take place faster.’ The outer shell of this primitive cell probably consisted of a bilayer of fatty acids, much like our current cells are also contained in a shell of lipids. Curiously, these primitive cells much resemble mRNA vaccines, the discovery of which earned a Nobel Prize in 2023. ‘It’s such a mind-triggering coincidence, I think, that the hope for the future of medicine resembles the very first moments of the origins of life.’
It’s such a mind-triggering coincidence that hopes for the future of medicine resembles the very first moments of the origins of life
‘These first primitive cells, called protocells, must have had a way to move towards light or nutrients to survive,’ Katsonis ponders. Understanding how this might have worked can be useful for these mRNA-based technologies. Katsonis studies the behaviour of protocells in her lab and discovered that the lipids in the encapsulation may have formed as an effect of the sun irradiating a layer of oil on water. This, by the way, is also useful knowledge to understand what happens to oil spills at sea, and to help clean them up. ‘But I did not think about applications when I started studying this,’ Katsonis adds. ‘I was just interested in the origins of life.’
Cleaning up oil spills
When oil spills at sea, a similar effect can occur as Katsonis observed in her lab: under the influence of sunlight, oil can transform into lipids. ‘To clean up an oil spill, it helps to understand these effects,’ the chemist explains. ‘For cleaning, surface active agents are used that split up the oil into smaller droplets. To do this, you need to know the composition, so that you can select the best surface active agents and the right amount.’
Katsonis currently has a setup in her lab in which she is testing the effect of light on an oil spill. ‘It really depends on the composition of the water. We are collaborating with the Royal Netherlands Institute for Sea Research (NIOZ), and they will send us samples of polluted water from the Rotterdam harbour. Then it’s up to us to see whether we can get these to clean themselves.’
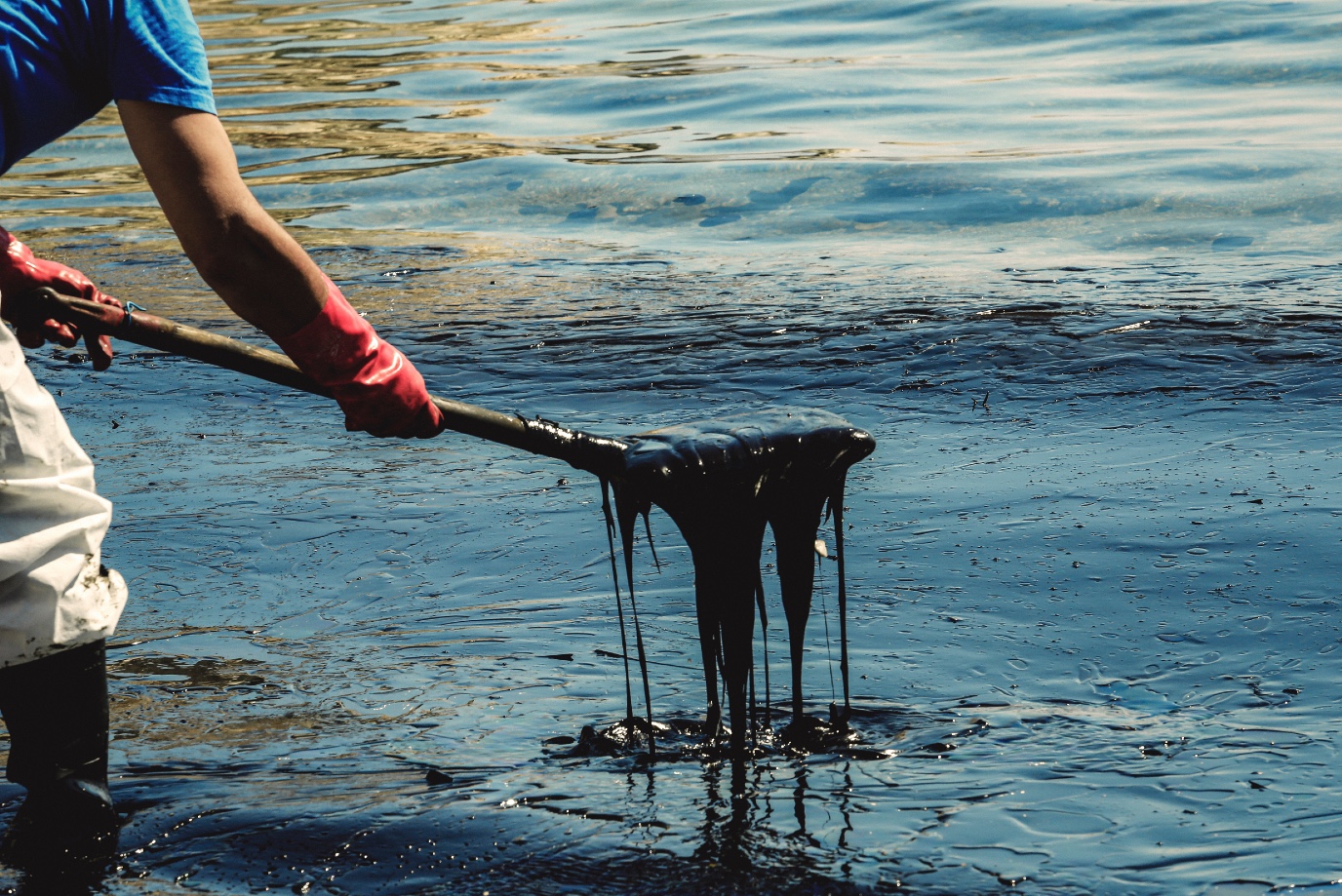
But how about the movement of protocells? ‘We think it may have had something to do with oil and water as well,’ Katsonis explains. ‘In Trinidad and Tobago, there is a lake that consists entirely of oil. Tiny water droplets can move through the oil lake and this water was encapsulated a long time ago. Isn’t that fascinating? Anyway, we don’t know yet exactly how an early life form may have moved. But I have some clues.’
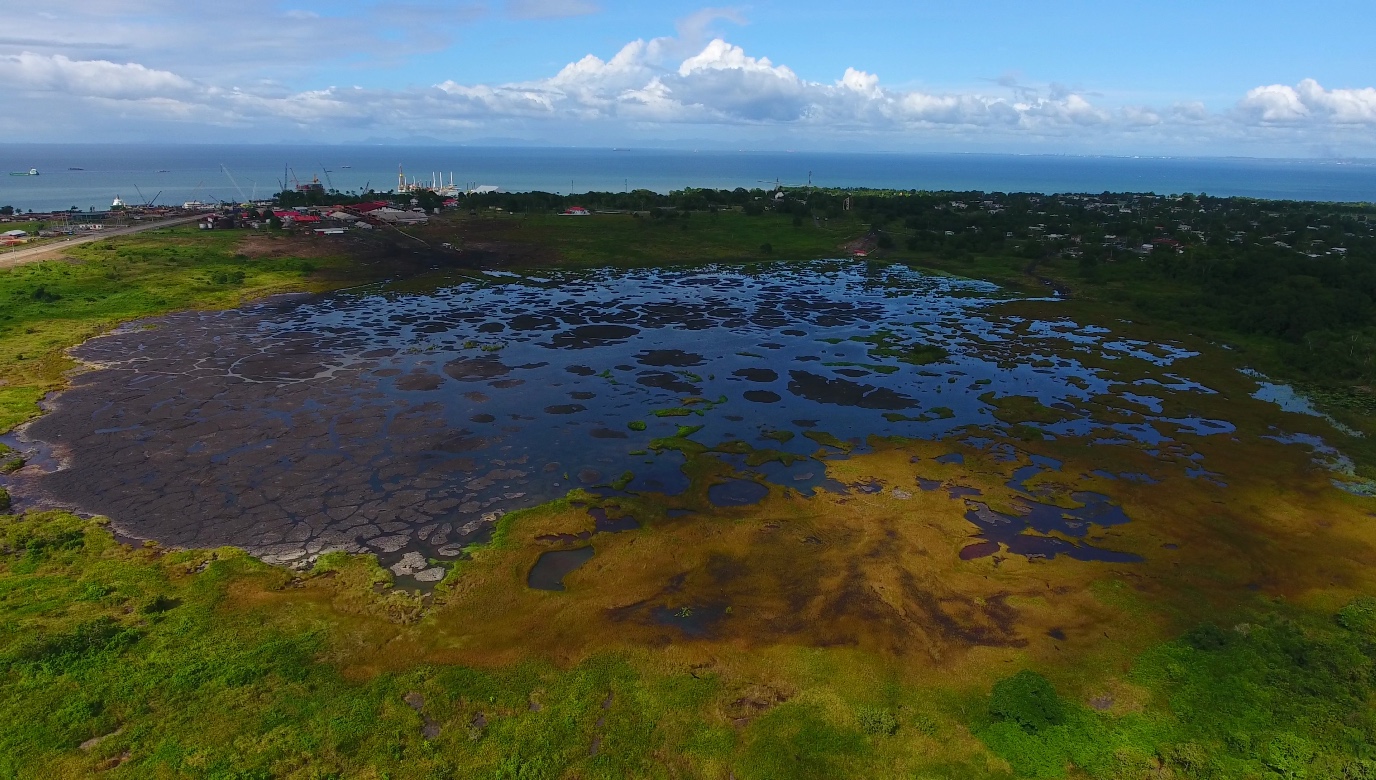
More information about Katsonis’ research from Ammodo and Katsonis’ research group.
Last modified: | 15 April 2025 2.12 p.m. |
More news
-
15 April 2025
1.5 million funding from Province of Groningen for innovative technology in the region
The University of Groningen will receive nearly 1.5 million euros in funding from the Province of Groningen to assist entrepreneurial academic researchers in developing innovative ideas into a startup.
-
15 April 2025
Nathalie Katsonis wins Ammodo Science Award 2025
For her pioneering research on molecular systems, Nathalie Katsonis receives the Ammodo Science Award for fundamental research 2025.
-
15 April 2025
Students test mini-satellites in municipality of Oldambt
Two student teams from the University of Groningen and Hanze University of Applied Sciences will test two self-built mini-satellites in the municipality of Oldambt on April 24.